Quantum Untangled - The layman's guide to qubit flavours
Here are the five main qubit types you need to remember.
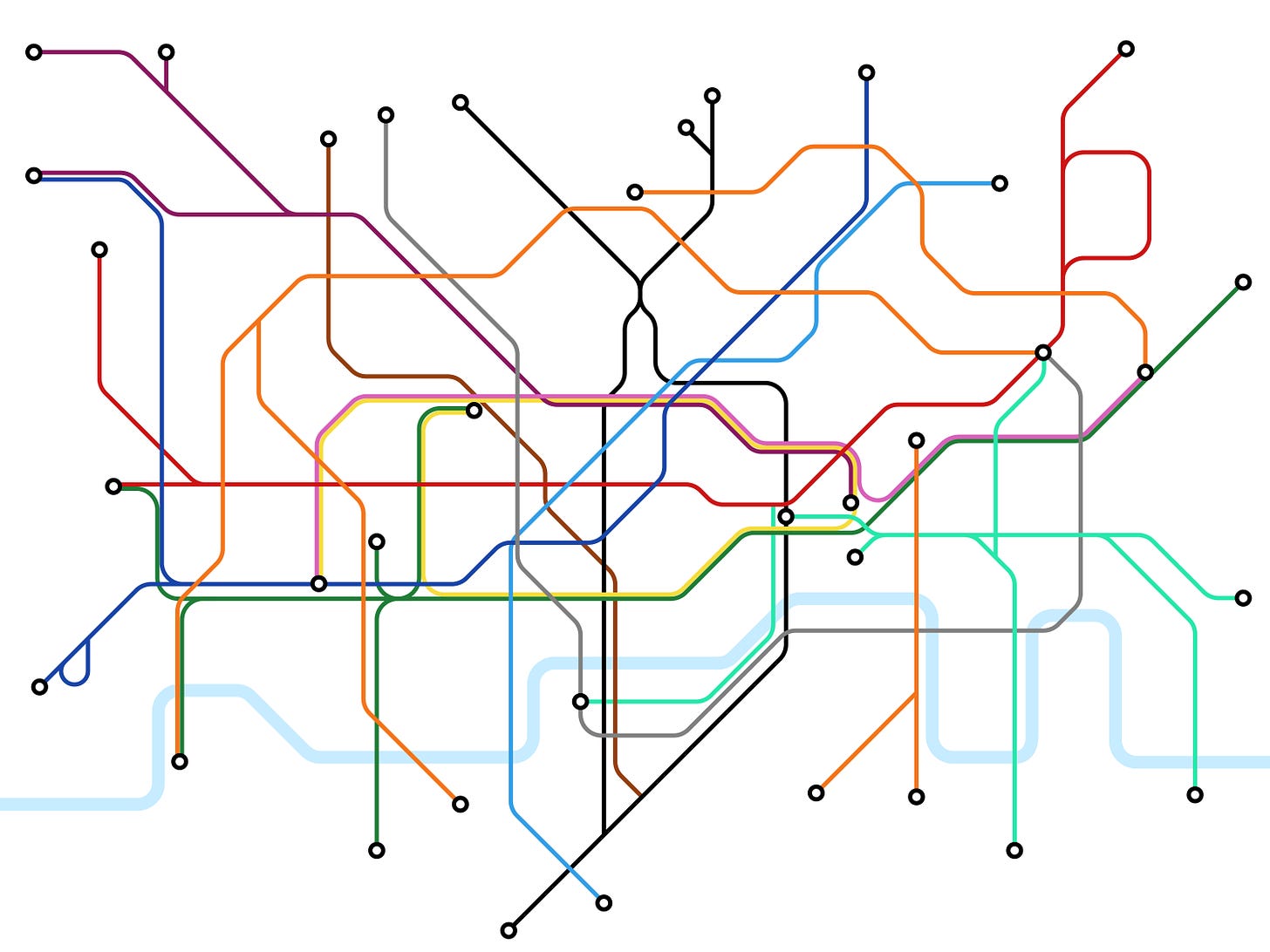
How do you get from King’s Cross station to Hatton Garden? Let me count the ways, as Shakespeare never, ever said in reference to London’s multivariate public transport system. You can, of course, use your legs during a brisk constitutional or cycle south skirting several pubs, clubs, parks and Victorian housing estates. Then there’s the No. 63 bus, or a black cab if you’re more financially adventurous. If public transport is your thing, then by all means use the Tube if you’re intent on wasting half an hour shunting down the Circle or Northern Lines – or, if you’re in possession of the requisite cheat codes, hop, skip and a jump over to nearby London St. Pancras to catch a Thameslink train that’ll get you to your destination in less than a jiffy.
In other words, there are more than a few ways to skin a cat. The same principle, the writer explains circuitously enough, extends to quantum computing (less so to cats, the field’s concerns about placing them in boxes notwithstanding.) Solving an intensely complex problem by harnessing quantum mechanics can be approached using many different types of qubit, each with their own trade-offs when it comes to resource allocation and stability. What isn’t clear yet is whether any single one of those will ‘win out,’ giving quantum its x86 moment — or whether each will survive, offering its own niche in the quantum space.
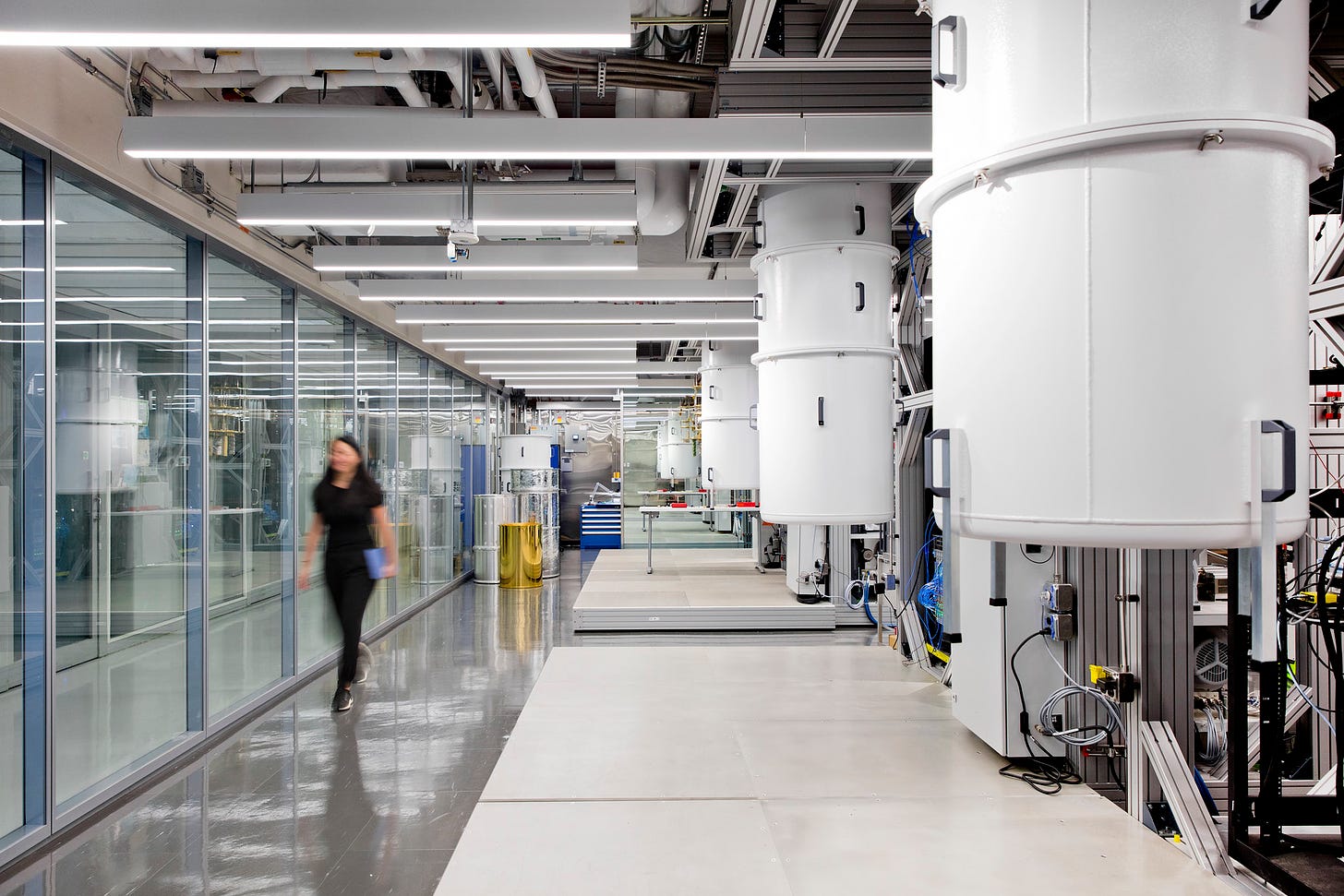
Superconducting qubits
One of the more mature forms of quantum technology, superconducting qubits are used by companies like IBM, Google and Finnish start-up IQM. Harnessing the properties of superconductivity, they are produced by cooling certain materials to near absolute zero. There are different types of superconducting qubits, each varying in its design. These include the flux, the phase and the transmon, the last of which is used by IBM. All superconducting qubits work by superconducting aluminium or, in some cases, niobium.
Dropping the temperature so low allows the quantum properties of these particles to manifest, which in turn permits the use of the semiconductors made out of the aluminium atoms. Microwave equipment is then used to interact with the qubits, all of which are stored on a chip similar to a classical computing CPU. This means that superconducting qubits can be fabricated at existing facilities and even integrated into other computing systems for hybrid quantum computing.
One of the biggest hurdles for superconducting qubits at scale, however, are their gigantic refrigeration requirements. As the machine scales and qubit numbers increase, so does the size of the refrigeration tower. IBM and others are working to solve this by connecting multiple chips or different machines together into something resembling a quantum supercomputer. The challenge therein is having the qubits share information across chips. Such machines are also heavily susceptible to noise, requiring greater degrees of error correction and mitigation, and have relatively low coherence times.
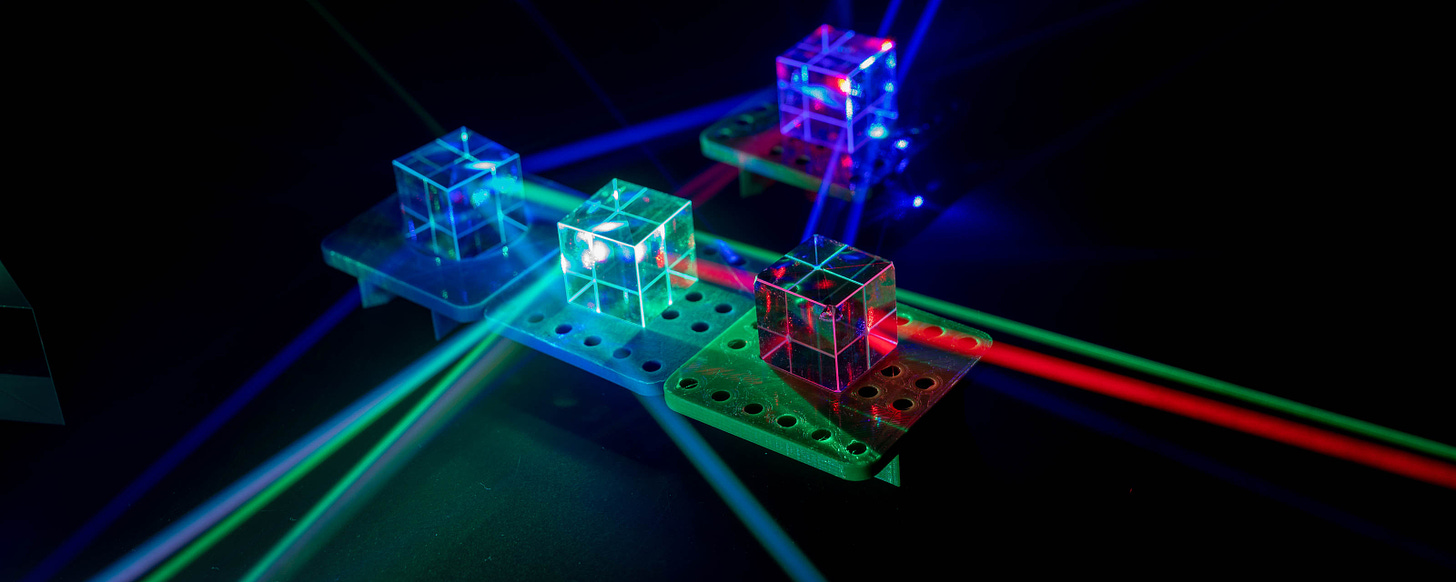
Photonics
In photonic quantum computing, the quantum information is carried on particles of light, known as photons. They don’t require the same degree of cooling as superconducting qubits and can therefore operate at room temperature. The photons are also more easily manipulated as they do not interact strongly with the environment or each other, which makes them less vulnerable to noise.
Photonic qubits also afford quantum researchers the opportunity to use a gate-based approach to quantum computing, performing operations on the qubits with beam splitters, phase shifters and mirrors. As such, these machines are harder to scale, as it is difficult to generate and detect single photons. Even so, as they operate at room temperature, photonic qubits can be more easily distributed to data centres or on-premises.
One of the big players in photonic quantum computing is the UK's ORCA. The company sold one of its machines to the UK’s Ministry of Defence last year, and have recently demonstrated their utility when working alongside GPUs to generate images and AI models.
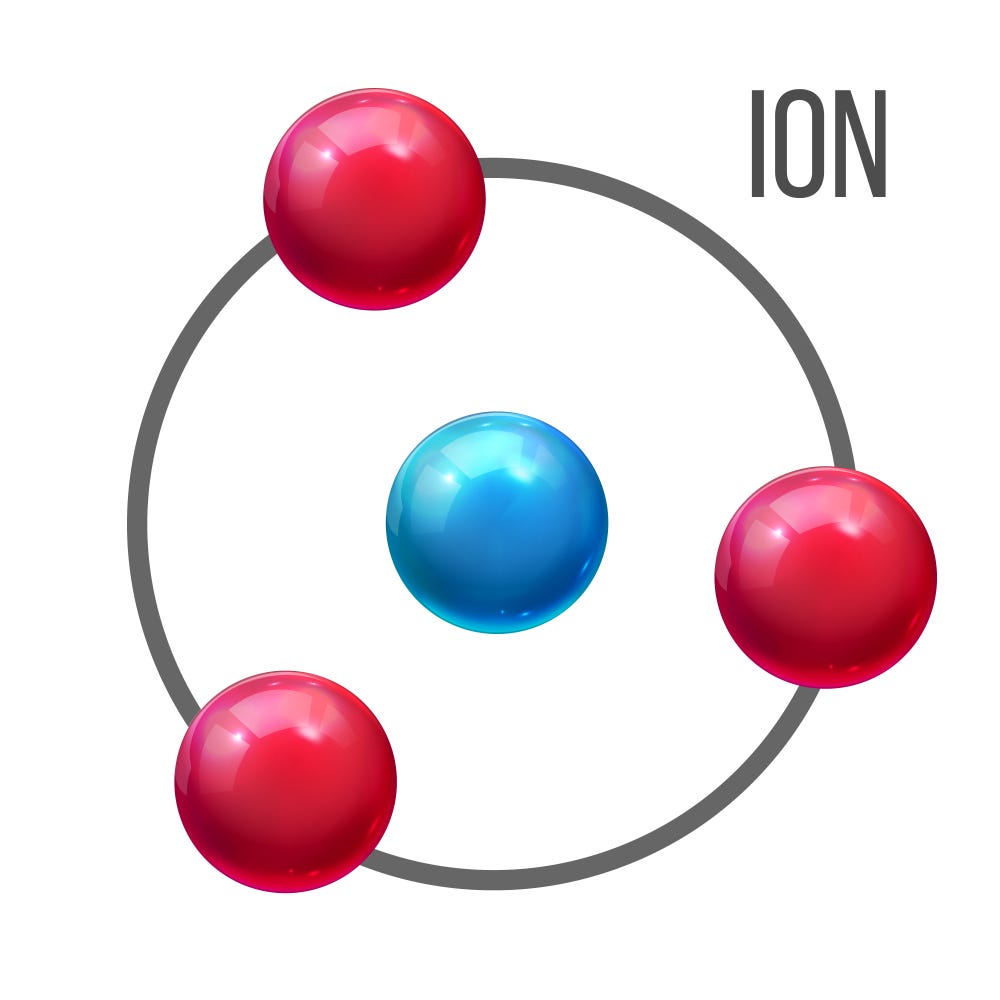
Trapped Ion
Trapped-ion technology is favoured by many up-and-coming quantum start-ups, including Quantinuum, IonQ and Oxford Ionics. In this technique, qubits are made from individual ions suspended in an electromagnetic field. Each ion is a qubit and interactions between them are controlled using laser or microwave radiation. That radiation is then used to execute logic gates by coupling the ground states of the ion to the motion of the ions. This induces entanglement, which allows the system to perform more complex computations.
They have particularly long coherence times, which is how long a system maintains its quantum state. It can be orders of magnitude higher than in superconducting qubits, reducing error correction requirements. It means quantum information can be stored and manipulated for longer allowing for high-fidelity operations. This has allowed for companies like Quantinuum to demonstrate high quantum volume and other industry metrics. The downside of trapped-ion is scaling as handling, controlling and maintaining a larger number of ions can be complex. Companies are starting to overcome this through improved traps and more efficient lasers.
While trapped-ions themselves don't need to be supercooled during operation and can be at room temperature, the surrounding machinery does need temperature control to maintain a high vacuum and stable electromagnetic field. The heat from the lasers also needs to be cooled (unless, of course, you like a good char on your lab coat of an evening.)
Diamond (Nitrogen-Vacancy Center)
One of the newer approaches to quantum, diamond quantum exploits the unique properties of a defect in diamond crystals known as the nitrogen-vacancy (NV) centre to form a qubit. NV centres form naturally when one atom in a pair of carbon atoms in the diamond lattice is replaced by a nitrogen atom and the other, well, isn’t, creating what is known as a vacancy. This, in turn, creates a ‘colour centre’ that can absorb and emit light. This is what gives the diamond used in quantum computing its light colour, compared with the clear colourless diamonds used in jewellery.
The NV also, usefully enough, possesses an electron ‘spin state’ that can represent a qubit. The spin state of an electron within the lattice can be up or down, and from there can be initialised, manipulated and read using laser light to process data. Like photonics, diamond qubits can maintain their quantum state at room temperature, leading to companies like Quantum Brilliance using them to produce edge-computing quantum devices that could, it claims, one day operate in driverless cars (see an earlier edition of the newsletter on quantum at the edge.)
Diamond qubits also suffer from similar scalability issues as other types of qubit. While a single NV centre acts as an excellent qubit, entangling multiple NV centres over a long distances can be a challenge. Control can also be complicated by different orientations with the diamond lattice, requiring the fabrication of synthetic diamonds.
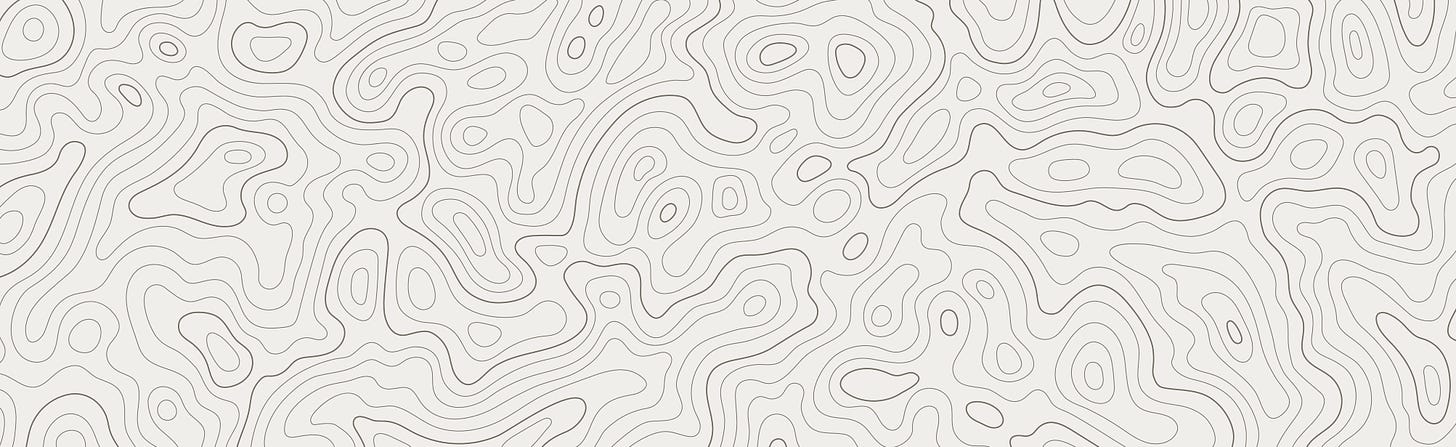
Topological qubits
Last but not least is the most experimental qubit of them all: the topological qubit. Still very much in their research stage, these qubits use a unique form of particle that can be braided and made resistant to noise and errors. They require a non-Abelian quantum phase of matter known as a topological state of matter. All of this was largely theoretical – that is, until breakthroughs by researchers from Microsoft and Quantinuum proved it was possible.
As this type of quantum computing was purely theoretical until last year, it isn’t clear yet what its full list of pros and cons are — frankly, it hasn’t been put to the test in the same way as superconducting, photonics and trapped-ion qubits have. However, the braided nature of the anyons used to carry data should allow for noise and error mitigation by default, requiring far fewer qubits to achieve utility.
It is likely topological quantum computers will still require supercooling like superconducting qubits, as the topological state of matter is very delicate and could be easily disturbed by thermal fluctuations. As for coherence times and scalability, that isn't clear yet. Anyons only exist in two-dimensional systems and have to be braided around one another to perform computations, an effect that so far has only been reproduced in two-dimensional electron gas stored in a strong magnetic field.
Despite all these uncertainties, topological qubits are seen by some as a viable route towards fault-tolerant quantum computing. As such, research proceeds apace into these elusive particles. For its part, Microsoft says it should have a topological quantum supercomputer ready in the next few years.
Partner content
How do we restore trust in the public sector? - The New Statesman
Defining a Kodak culture for the future - The New Statesman
Brands must seek digital fashion solutions - Tech Monitor
Green bonds and the urban energy transition - Capital Monitor